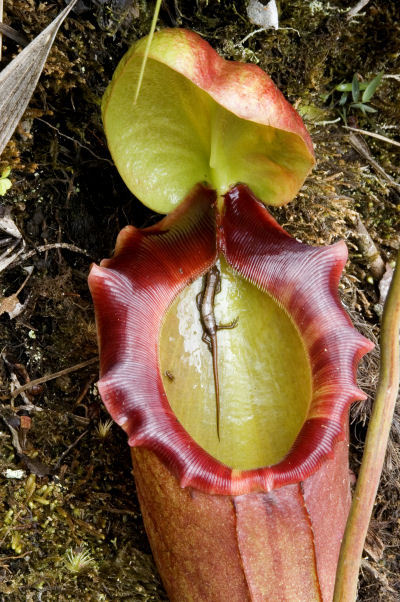
As of 2013, kratom has been studied in cells and in animals, but no clinical trials have been conducted in the United States.[6] The U.S. Drug Enforcement Administration (DEA) said in 2013 that there is no legitimate medical use for kratom.[13] and in 2019, the U.S. Food and Drug Administration (FDA) said that there is no evidence that kratom is safe or effective for treating any condition, and that there are no approved clinical uses for kratom.[11]
Kratom leaves are commonly used by chewing, as a tea, powdered in capsules or pills, or extracted for use in liquids.[6] Kratom is rarely smoked.[21] Different varieties of kratom contain different relative proportions of alkaloids such as Mitragynine.
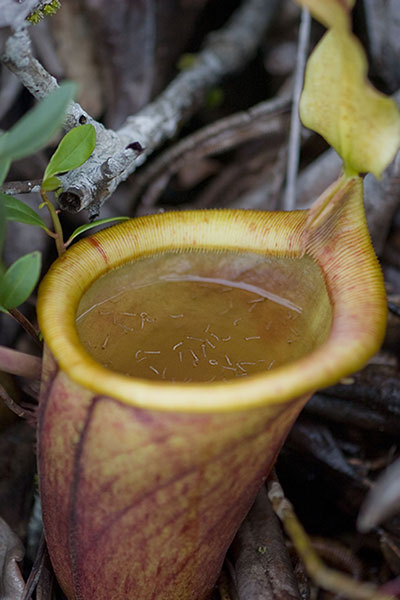
While many Nepenthes species are generalists in what they capture, at least one,
N. albomarginata, has specialised and almost exclusively traps termites and produces nearly no nectar.
Nepenthes albomarginata gains its name from the ring of white trichomes directly beneath the peristome. These trichomes—or “hairs”—are palatable to termites and will attract them to the pitcher. In the course of collecting the edible trichomes, hundreds or thousands of termites will fall into the pitcher.
Symbioses
N. bicalcarata provides space in the hollow tendrils of its upper pitchers for the carpenter ant Camponotus schmitzi to build nests. The ants take larger prey from the pitchers, which may benefit
N. bicalcarata by reducing the amount of putrefaction of collected organic matter that could harm the natural community of infaunal species that aid the plant’s digestion.
N. lowii has also formed a dependent relationship, but with vertebrates instead of insects. The pitchers of
N. lowii provide a sugary exudate reward on the reflexed pitcher lid (operculum) and a perch for tree shrew species, which have been found eating the exudate and defecating into the pitcher. A 2009 study, which coined the term “tree shrew lavatories”, determined between 57 and 100% of the plant’s foliar nitrogen uptake comes from the faeces of tree shrews.
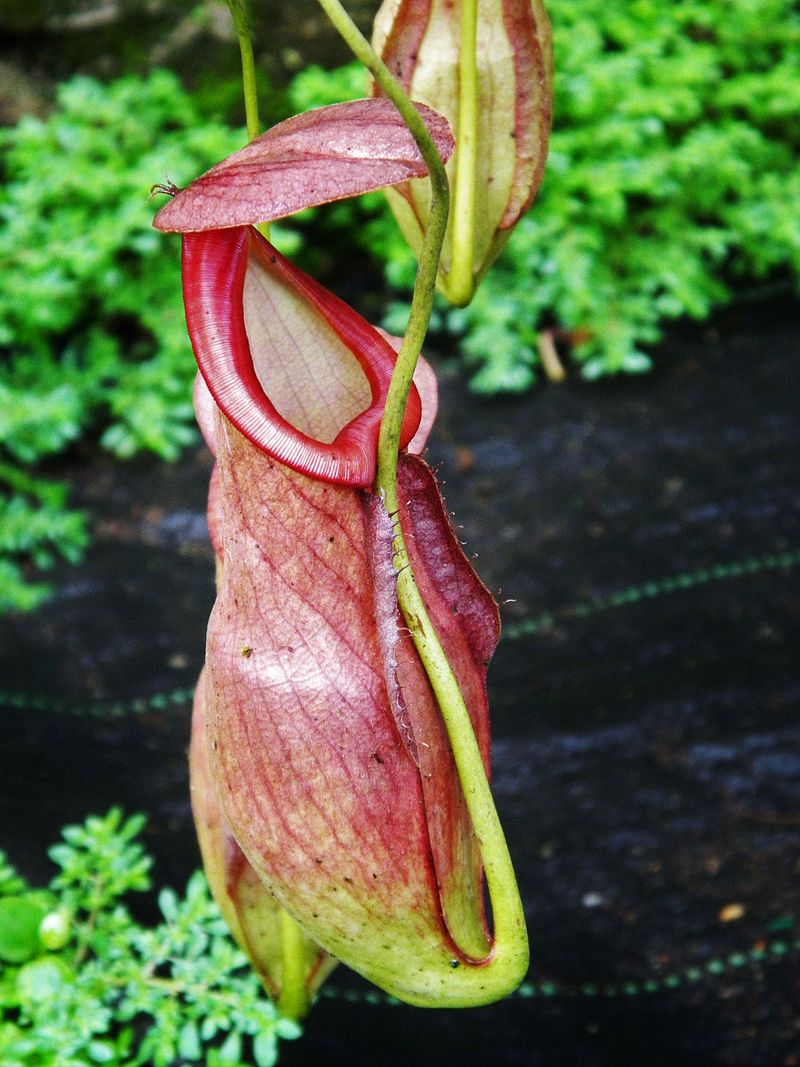
Another study showed the shape and size of the pitcher orifice of N. lowii exactly match the dimensions of a typical tree shrew (Tupaia montana). A similar adaptation was found in N. macrophylla, N. rajah, N. ampullaria, and is also likely to be present in
N. ephippiata.
Similarly,
N. hemsleyana, which is native to Borneo, has a symbiotic partnership with Hardwicke’s woolly bat. During the day, a bat may roost above the digestive fluid inside the pitcher. While a bat is inside, it may defaecate, and the plant can get nitrogen from the droppings.
Antimicrobial properties
Nepenthes digestive fluids are sterile before pitchers open and contain secondary metabolites and proteins that act as bactericides and fungicides after the pitcher opens. While the digestive fluid is being produced, the pitcher is not yet open, so there is no chance of microbial contamination. During pitcher development, at least 29 digestive proteins including proteases, chitinases, pathogenesis-related proteins and thaumatin-like proteins are produced in the pitcher fluid. In addition to breaking down prey, these can act as antimicrobial agents. When the pitchers open, the fluid is exposed to bacteria, fungal spores, insects and rain.
Often pitchers have a lid that covers the trap, excepting a few (e.g.
N. lowii, N. attenboroughii and
N. jamban), preventing rain water from entering. The lid inhibits rainwater from diluting the digestive fluid. Once the bacteria and fungi enter the fluid, secondary metabolites are produced in addition to antimicrobial proteins. Naphthoquinones, a class of secondary metabolite, are commonly produced, and these either kill or inhibit the growth and reproduction of bacteria and fungi. This adaptation could have evolved since Nepenthes plants that could produce secondary metabolites and antimicrobial proteins to kill bacteria and fungi were most likely more fit. Plants that produced antimicrobial compounds could prevent loss of valuable nutrients gained from insects within the pitcher. Since Nepenthes cannot digest certain bacteria and fungi, the bactericides and fungicides allow plants to maximize nutrient uptake.